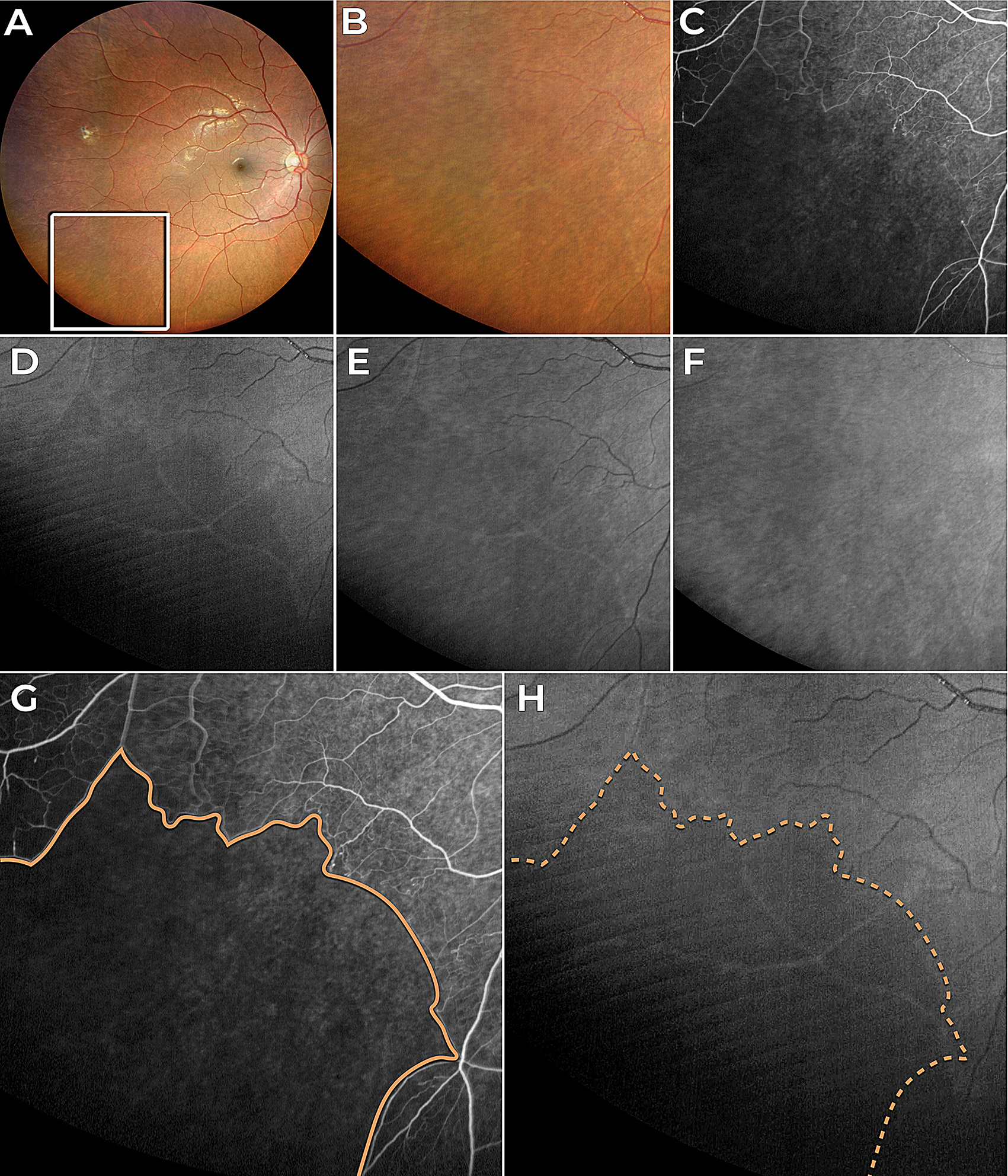
Our analysis of five cases demonstrated a correspondence between perfusion defects, identified by FA and OCTA, and hyporeflectant areas in BLR images. Also, the study was able to identify the absence of the INL and OPL at these hyporeflectant areas in all five cases with available OCT scans.
This discussion will elaborate on the anatomical and physiological underpinnings that might support this observation, integrating knowledge from the available literature and the inherent properties of retinal layers as they interact with blue light. The findings emphasize the utility of non-invasive imaging techniques in assessing retinal vascular health, supported by the anatomical and physiological characteristics of retinal layers and their interaction with blue light.
Monochromatic fundus imaging using different wavelengths of light interacts with the retina in various ways, highlighting specific structures and revealing details not visible in CF images. Understanding these interactions is crucial for optimizing diagnostic imaging in ophthalmology [1].
The GLR provides excellent overall retinal contrast. It is partially reflected by retinal pigmentation and absorbed by blood, making it particularly effective for viewing retinal blood vessels, hemorrhages, drusen, and exudates [12]. In contrast, the RLR is instrumental in visualizing deeper structures within the eye. Red light wavelength penetrates deeper into the retina and choroid, revealing more of the choroidal pattern. This is useful for visualizing pigmentary disturbances, choroidal ruptures, choroidal nevi, and choroidal melanomas [12].
Blue light wavelength enhances the visibility of the anterior retinal layers, which are typically nearly transparent under white light. This wavelength is highly effective in highlighting semitransparent structures due to its high scattering properties [1]. The blue light is strongly absorbed by macular pigment such as lutein and zeaxanthin, hemoglobin within the blood vessels, and melanin at the level of the RPE and choroid, the latter creating a dark background that accentuates specular reflections and scattering in the anterior retinal layers [1].
Historically, this method is associated with increasing visibility and aids in the identification of the retinal nerve fiber layer, internal limiting membrane, retinal folds, cysts, and epiretinal membranes [1]. However, recent evidence shows that the benefits of BLR are not limited to these conditions, playing an important role in the evaluation of other retinal conditions such as Type 2 Macular Telangiectasia [13], subretinal drusenoid deposits [14], and central serous chorioretinopathy [15].
Previous research, including our preliminary study, has shown the blue channel’s ability to identify retinal arteriolosclerosis, sclerosed venules, and areas of retinal ischemia [5,6,7,8]. Hyporeflectant areas on BLR obtained through confocal scanning laser ophthalmoscopy (cSLO) showed a high correlation when compared to FA in patients with diabetic retinopathy (DR) [5, 7] and retinal vein occlusion (RVO) [5]. Also, an association between retinal thinning and disorganization in OCT was found [7]. This research also noticed that the area of nonperfusion appeared larger on FA than BLR [7].
In attempting to explain the possible cause of hyporeflectant areas in the non-perfused retina, it was suggested that this phenomenon may occur due to reduced blood flow, as the authors proposed that blue light is reflected by red blood cells (RBC), and the absence of RBC would result in a hyporeflectant appearance [5]. However, blue light is not reflected by RBC, but rather absorbed by the hemoglobin present in RBC, which accounts for the hyporeflectance of blood vessels and hemorrhages in the images captured by this method [1].
Another recently raised hypothesis was the reduction in thickness and disorganization of the retina, observed in OCT, as a possible cause. However, since only a few cases were evaluated, the authors did not delve deeply into this topic [7]. The possibility of a reduction in nerve fiber layer thickness secondary to ischemic insult leading to decreased reflectance was also considered. Nonetheless, this was ruled out, as the hyporeflectant areas did not follow the anatomical distribution of the nerve fiber layer [7].
In the current study, we observed hyporeflectance in the retina on the BLR in non-perfused regions. Following ischemic damage caused by retinal non-perfusion, particularly in the DCP, the retina clinically reacts in the initial stages with grayish-white patches that return to normal coloration within a few weeks. On OCT, these areas appear hyper-reflective in the acute phase, progressing to reduced reflectivity and thinning (atrophy) of the inner retinal layers. When the deep capillary plexus is exclusively affected, the INL and OPL layers undergo atrophy in the late stages.
The findings from OCT and OCTA, correlated with the BLR findings in this study, suggest that hyporeflectant areas occur due to atrophy of the INL and OPL layers of the retina. This is supported by the following observations: (1) In all presented cases, structural OCT showed a correlation between hyporeflectant areas and the absence of INL and OPL. (2) Even in the case with normal perfusion of the SCP, as seen on OCTA, which displayed INL and OPL atrophy on structural OCT due to ischemia in the DCP, the hyporeflectant areas in the BLR correlated with the areas of INL and OPL atrophy.
These observations lead us to consider that the INL and OPL layers, either together or individually, play a fundamental role in the reflection of blue wavelength light. To the best of our knowledge, this is the first study that correlates BLR to specific retinal structures.
Given these findings, we believe that, just as the near-infrared (NIR) wavelength used in cSLO NIR reflectance imaging has a significant portion of its energy reflected by the interdigitation zone and consequently has the potential to facilitate the identification of diseases such as acute macular neuroretinopathy and hydroxychloroquine-induced drug toxicity, BLR has a specific interaction with the INL and OPL layers and has the capability to identify changes in these layers.
This unprecedented information is particularly noteworthy as it illustrates the potential for expanding the indications of the technique to conditions beyond diabetes and venous occlusions [5, 7, 8]. Our current study expands these preliminary observations by demonstrating the applicability of BLR imaging across a range of retinal non-perfusion scenarios.
Previous studies evaluating non-perfused areas using blue light reflectance were conducted with image acquisition via cSLO [5, 7], raising the question of whether these findings would be reproducible with other blue-light imaging methods [7]. The findings of the present study elucidate this issue, demonstrating the reproducibility of these results by decomposing the RGB channels from a CF image.
Unlike the cSLO system, which is expensive and not widely accessible, fundus photography is a widely used and cost-effective imaging technique. The use of digital filters to separate image channels is well-established and can be easily implemented without additional cost using embedded software in several retinal cameras or free software like ImageJ. For instance, in ImageJ, the command “Image -> Color -> Split Channels” allows for the separation of the BLR, enabling the identification of non-perfused areas [16].
BLR imaging may offer clinical utility in settings where access to OCT/OCTA is limited or for patients who cannot undergo contrast-enhanced imaging. The ability to identify non-perfused areas using readily available retinal cameras makes this technique particularly valuable in such contexts. Our findings suggest that BLR imaging can complement angiography by indicating areas of DCP ischemia, providing a more comprehensive evaluation of the retinal vascular meshwork without relying on OCT.
We highlight the critical role of ischemia in retinal diseases and underscore the positive impact of identifying such biomarkers. The potential of BLR imaging to detect DCP ischemia, even when SCP perfusion appears normal, presents a significant advancement in diagnosing retinal vascular diseases.
Furthermore, the observations of the present study explain the possible reason why a previous study noted a larger area of non-perfusion in fluorescein angiography compared to the BLR image [7]. Considering that hyporeflectance in the BLR occurs after the atrophy of the INL and OPL layers, and that this atrophy takes weeks to develop following the ischemic damage caused by retinal non-perfusion, it is plausible to speculate that recent hypoperfused areas seen in FA may not correspond to hyporeflective areas in the BLR. For instance, in Fig. 1, non-perfusion areas on FA appear larger than those seen in BLR images, which may be due to the time lag between ischemic insult and the development of retinal thinning detectable by BLR imaging. However, future research with longitudinal follow-up of these patients is necessary to confirm this hypothesis.
The findings of this study, particularly the correlation between hyporeflectant areas in BLR images and the absence of identifiable INL and OPL in structural OCT B-scans offer valuable insights into the complex interaction of blue light with retinal tissue. This process is dictated by the optical properties of blue light and the unique anatomical and biochemical characteristics of the inner retinal layers. Each layer possesses the inherent property of reflecting incident light, known as reflectivity, with blue light undergoing significant scattering due to its shorter wavelength and higher energy. This scattering is influenced by microstructural components, such as cylindrical organelles, and the thickness, density, and organization of the retinal architecture. The retina’s distinct structural and cellular layers generate specific reflectivity patterns, with high reflectivity seen in synaptic layers like the OPL, while nuclear layers such as the INL demonstrate lower reflectivity [17].
The OPL plays a crucial role in the retinal architecture and function by containing synapses among and between retinal photoreceptors, horizontal cells, and bipolar cells. Desmosome-like attachments called synaptic densities are located within the arrangement of interwoven [17]. These synaptic densities are seen as a series of dashed lines on light microscopy and resemble a discontinuous membrane, termed the middle limiting membrane, that might characterize resistance to the penetration of blue light. In retinal imaging, the presence of the OPL can influence the distribution and reflection of light, contributing to the overall reflectivity patterns seen in BLR.
Despite the strong theoretical basis suggesting the OPL as the most likely structure related to BLR, every analyzed image showed that the hyporeflectant areas consistently lacked both OPL and INL layers. Our study was unable to determine whether a single layer or a combination of both is primarily responsible for reflecting and scattering blue light.
While BLR imaging offers several advantages, it is important to acknowledge the broader limitations of this study. The sample size is relatively small, with only one or two cases representing each retinal disease, which restricts the generalizability of our findings and does not account for varying severities or stages of the diseases. Additionally, the correlation between different imaging modalities was not subjected to statistical analysis; instead, it was evaluated qualitatively. Furthermore, BLR imaging itself is subject to limitations, including interference from media opacities (e.g., cataracts), blue-light filtering intraocular lenses, and prior use of fluorescein eye drops, which can create image artifacts [18]. These factors can affect the clarity and accuracy of BLR images, necessitating careful consideration when interpreting results [12]. Discoloration in ischemic areas can be seen in color fundus images, and while challenging, some retina specialists can detect these changes. We emphasize that this study did not aim to evaluate the sensitivity nor specificity of the detection of these findings using BLR, but rather to show that the identification of non-perfusion is within a range of retinal non-perfusion scenarios.
In addition, BLR acquired from color fundus photography is valuable for detecting retinal nerve fiber layer defects in glaucomatous patients, often outperforming red-free imaging [19, 20]. However, it should be complemented by other modalities like OCT and FA to assess deeper retinal layers and differentiate between ischemic and non-ischemic retinal damage.
This study reinforces the evidence, through OCT, OCTA, and angiographic correlation, that the BLR can effectively identify areas of retinal non-perfusion in a non-invasive manner. Future research should explore the interaction of different light wavelengths with retinal tissue to enhance our understanding of retinal anatomy and physiology. This could lead to improved diagnostic capabilities and potentially uncover new therapeutic targets. In conclusion, BLR imaging presents a promising, cost-effective, and non-invasive method for detecting retinal non-perfusion, with significant implications for clinical practice, particularly in resource-limited settings and for patients unable to undergo contrast-enhanced imaging.
Leave a Reply